Measuring the Impossible at Oak Ridge National Laboratory
Researchers are getting a deeper understanding of fusion reactions with the help of spectroscopy and ICCD cameras
Fusion research has been conducted continuously for more than 70 years, but recent milestones like net energy gain and the world’s largest experimental reactor coming online have put fusion research squarely into the spotlight once again. As an emission-free source of power without long-lasting nuclear waste, the promise of changing the future of energy has brought private fusion companies into a field once entirely composed of public researchers, potentially putting fusion power in our near (or at least nearly near) future.
But what largely drives today’s headlines are tests: early evidence that a fusion-powered future is possible. Many of those measurements are being perfected at the Oak Ridge National Laboratory.
What is Oak Ridge National Laboratory?
The Department of Energy’s Oak Ridge National Laboratory promises to “solve big problems,” which its scientists have been doing since it was first created in 1947 as part of the Manhattan Project. Its decades of discoveries include promethium and the Y chromosome, along with energy milestones like the first nuclear power produced as electricity. Today, the lab houses the most powerful supercomputer in the world—tasked with creating once-unimaginably complex simulations in a variety of scientific fields, from astronomy to advanced materials. And, of course, fusion power.
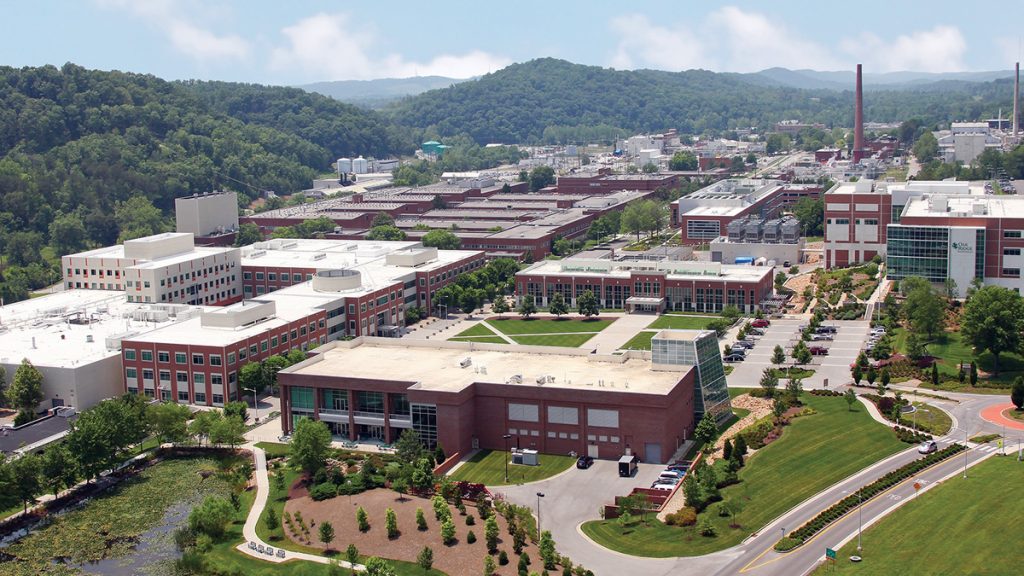
Under the Burning Plasma Foundations Section, Oak Ridge’s Diagnostics and Control Group develops and validates the capabilities needed to understand and control what’s happening inside a fusion device. The group works on a variety tokamak reactor projects, from the domestic DIII-D National Fusion Facility to the international ITER project, improving the measurements that literally define fusion research. The world may be watching, but they’re the ones with their eyes on the reactions.
Too hot to handle.
Measuring the plasmas at the center of fusion power isn’t quite staring directly into the sun, which is itself an immense fusion reaction. But it’s close.
Plasma reactions can reach temperatures of 150 million degrees Celsius, which makes direct contact impossible. And even without anything as dramatic as melting components into liquid metal, irradiation can still be potentially catastrophic for measurement devices. The radiation released by a fusion reaction causes a variety of effects on nearby materials, including transmutation—modifying the materials’ fundamental structures.
Irradiation can also cause material to harden and become more brittle, making them prone to breaks. It can even increase the conductivity of insulators, undermining their usefulness, or add an electromagnetic force to electrical cables that’s comparable to their useful signal.
Optical components like fiber optics aren’t immune either. Transparent materials can acquire color or become opaque if they’re sufficiently irradiated. But optical solutions are still a useful way of keeping the equipment away from the reaction and gathering essential information.
Scientists at Oak Ridge are having to invent the research techniques that will allow us to understand what’s happening in the hostile environment of a fusion reactor. Their first test bed, the Proto-MPEX helped set the foundation for the science of plasma material interactions (PMI). The device also supported the development of research techniques for the study of materials capable of withstanding the extreme conditions inside fusion reactors. From the beginning of operations in May 2014 until its retirement in 2021, scientists executed close to 30,000 plasma pulses in the Proto-MPEX, yielding data that results in more than 50 publications.
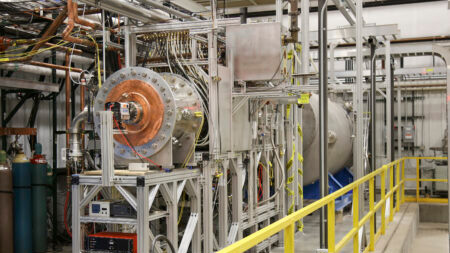
Over the next few years, scientists and engineers will be working on its sequel, the MPEX. This new system will be designed to investigate our most urgent remaining questions about plasma materials interactions.
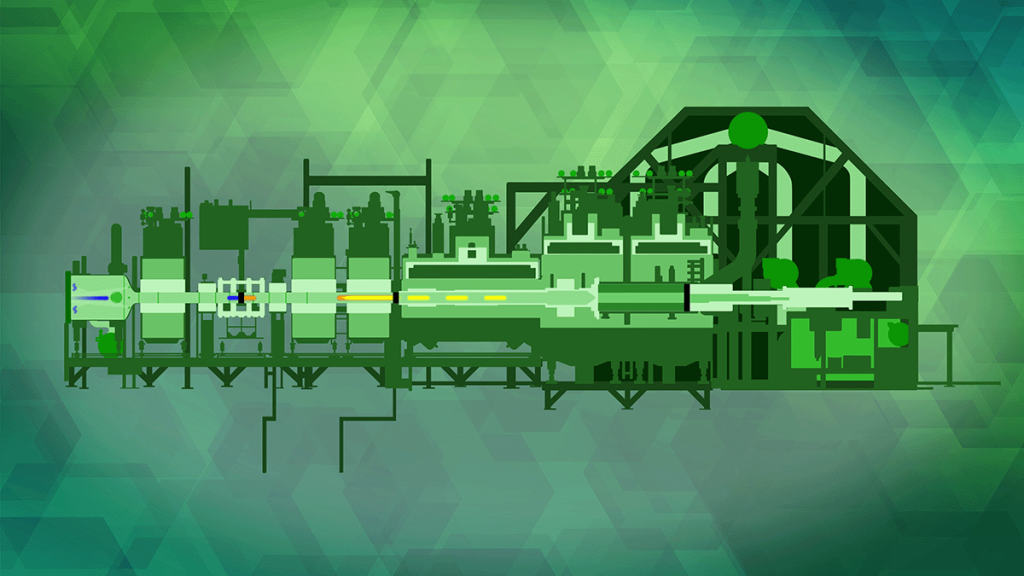
Bring in the lasers
Thomson scattering is an “active” optical method of measuring the plasma reaction. Experimenters direct repeated pulses from a laser into a plasma, then measure the photons that are scattered through it. This lets them measure electron temperature and density throughout the plasma via spectral bands, but they need to account for the active interference from the laser itself.
Because the Diagnostics and Control Group includes a wide range of projects whose experiments often have very different parameters, they have created a compact, portable system for observing Thomson scattering. Using both IsoPlane®320 spectrographs and PI-MAX® 4 ICCD/emICCD cameras, they were able to get both flexibility and precision.
The high temperatures generated at the beginning of reaction gives a continuum emission that masks the distinct wavelength signatures of individual atom and ion species. To identify them, the spectrograph must offer high resolution and fast performance to distinguish those narrow peaks as the plasma quickly cools.
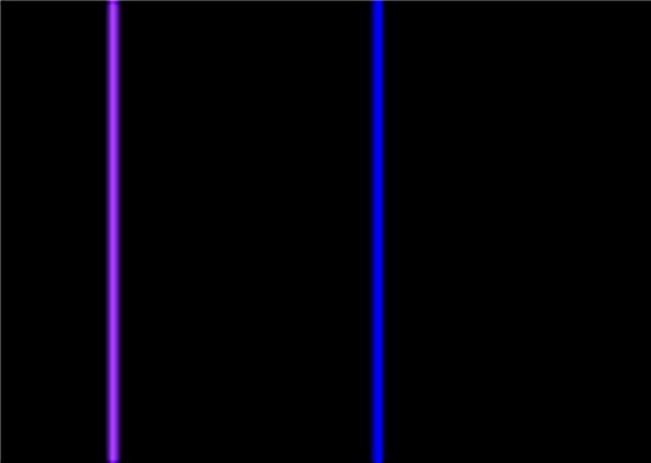
Flexibility
It isn’t just the range of their work that requires the Diagnostics and Control Group to stay flexible: temperatures themselves range from hundreds of millions of degrees down to even relatively “cold” plasmas at 1,000 times room temperature.
Thomson scattering systems can use hundreds of input channels over several stages, so it helps to have a compact, scalable solution that easily can be expanded to host more measurement channels. This setup uses multiple fiber optic inputs to address different locations in the experiment, while the IsoPlane spectrometer’s triple-grating turret lets experimenters easily change the diffraction gratings that separate light into individual wavelengths.
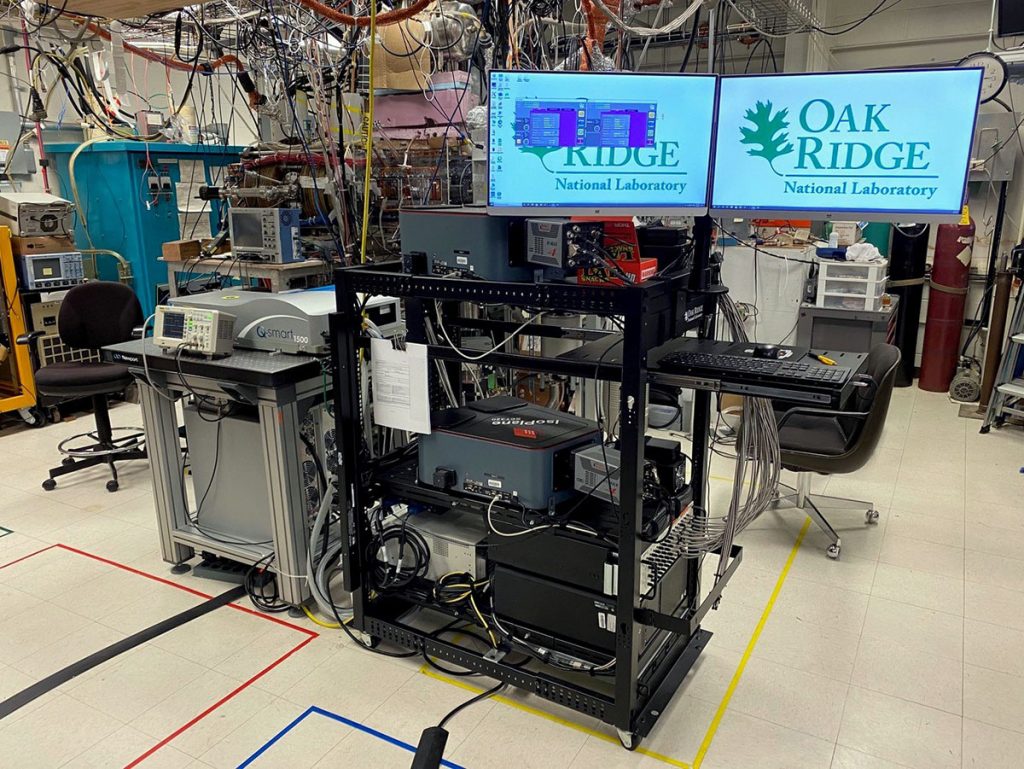
Precision
The use of a CCD imager integrated an intensifier allows for six times more light to pass between the imaging intensifier and the detector. At the beginning of the plasma process, high temperatures mask the sharp lines of individual atomic “species.” The PI-MAX 4 camera offers precision gating to <500 picoseconds, which makes it possible to accurately see the laser signal against a bright plasma background.
And while most lenses have a degree of astigmatism, the IsoPlane spectrometer doesn’t. Instead, it has has a collimating mirror, a dispersive grating, and a focusing mirror. This means it doesn’t have to correct for the distortions that would be caused by the shape of its lens.
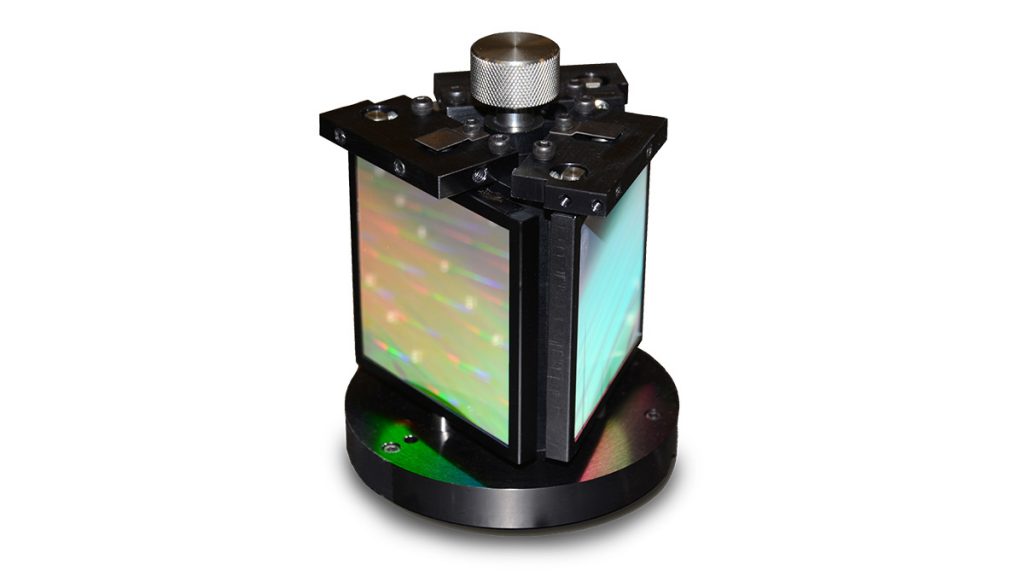
The future depends on what’s happening this (micro)second
All of the possibilities for fusion power we’ve discussed depend on the accuracy of experimental measurements. And understanding a variety of experiments will require flexible approaches in a growing industry. The precision measurements this equipment makes are now being fed back into another Oak Ridge’s core competencies: supercomputing. The vast amount of data is being used by the Plasma Theory and Modeling Group to inform its state-of-the-art simulation and modeling capabilities to resolve critical issues and to inform the design and operation of future fusion devices. These minute measurements will help us paint the broadest strokes of the future.